But can only our planet boast of this grand spectacle? Can, if not the indigenous people, then future colonists, for example, Mars or the moons of Jupiter, observe something like this?
What does it take for auroras to appear on any planet?
By definition, auroras are the luminescence of the upper atmospheric layers of planets with a magnetosphere due to their interaction with charged particles of the solar wind.
So we need:
1. The solar wind, which is a stream of charged particles - protons, electrons, helium nuclei, etc. - It is always present in the entire solar system.
Planets or their satellites:
2. The atmosphere with the atoms of which the solar wind will interact.
3. A magnetic field that directs charged particles to a specific region of the planet (not necessarily to the polar, - the angle between the magnetic axis and the axis of rotation of the planet can be significant.)
Let's see how it works on Earth.
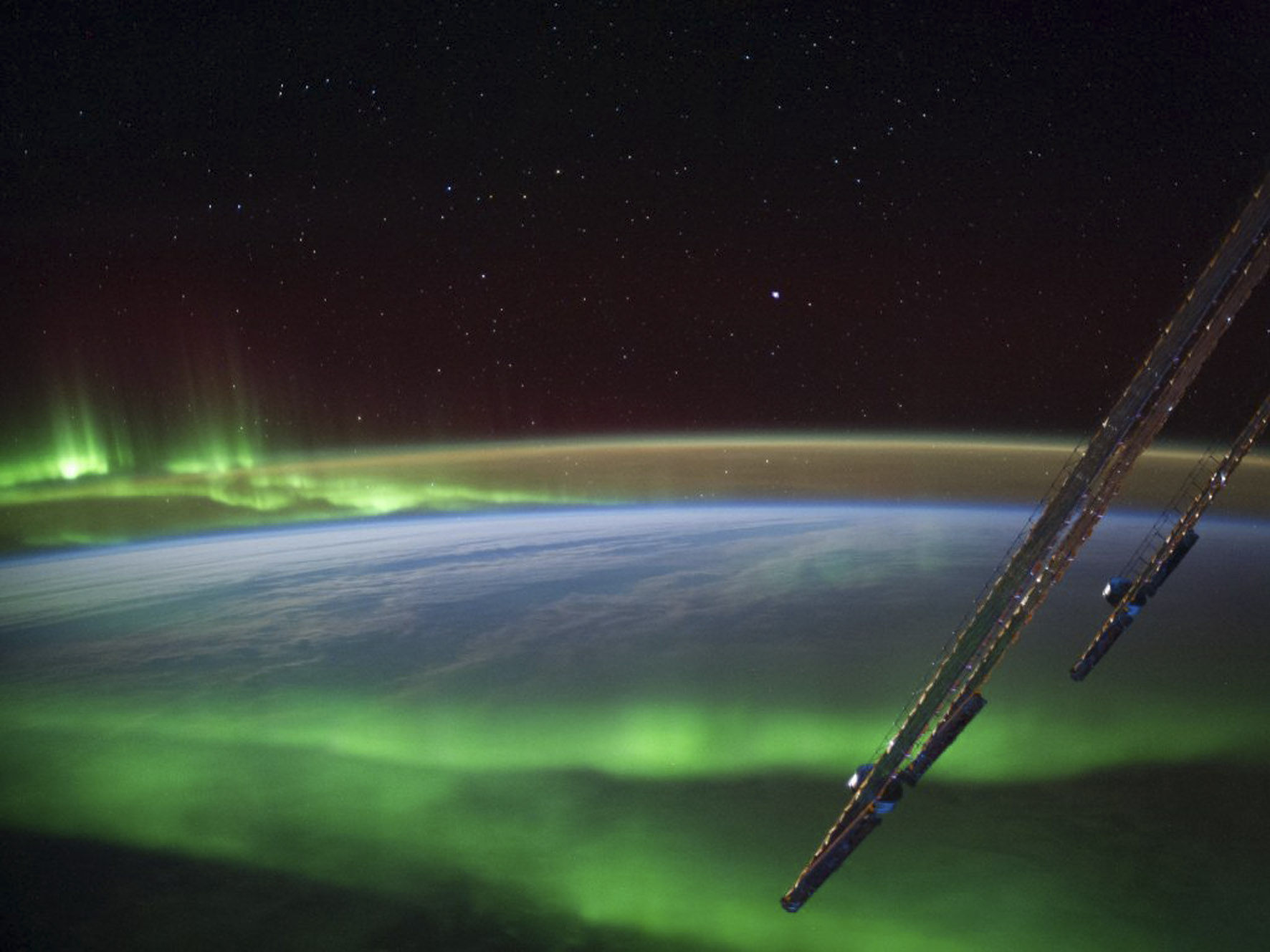
Land
The earth can be considered as a large magnet, the south pole of which is located near the north geographic pole, and the north is near the south. The geomagnetic lines of the Earth are slightly compressed from the side of the Sun due to the pressure of the solar wind and are pulled in the opposite direction, forming a magnetospheric tail at the Earth.
And how do solar wind particles behave when interacting with the planet’s magnetosphere? - In near-Earth space, everything happens as with a supersonic plane. - The flow of the solar wind at a supersonic speed (400-700 km \ sec) runs onto the magnetosphere of the planet, resulting in the formation of the so-called head shock wave. - (The speed of the solar wind in the Earth’s orbit is about 10 times the speed of sound in near-Earth plasma.)
A head shock wave is thus a magnetic obstacle that deflects charged particles of the solar wind along trajectories around the planet. Flying on it, most charged particles simply flow around the magnetosphere.
Some part of the solar plasma falls into the magnetic traps of the Earth’s radiation belts - it is difficult for charged particles to move across the lines of force and they simply wound around them and can hang from pole to pole for decades.
And still part freely penetrates into the polar ionosphere through the polar cusps - funnel-shaped areas expanding from the Earth to the magnetopause, resulting from the interaction of the solar wind and the Earth's magnetic field.
Through the cusps, particles of the solar wind “spill out” into the upper layers of the planet’s atmosphere in two regions at high latitudes.

Earth's magnetosphere
These regions are two ovals (in the northern and southern hemispheres), approximately 20 ° from the geomagnetic poles at night and 10 ° from the daytime. The length of these oval areas in latitude is only a few hundred kilometers.
With an intense magnetic storm, the oval shifts strongly towards the equator.
And if during periods of a quiet Sun the intensity of auroras is, to put it mildly, small, the matter is aggravated during solar activity. Emissions of coronal mass (plasma from the corona of the sun) greatly increase the intensity of the solar wind.
Magnetospheric substorms are added to the fire. During them, in the geomagnetic tail (on the night side of the Earth), the lines of force of the interplanetary magnetic field and the geomagnetic field of the Earth reconnect. As a result, the line topology changes, the explosively released energy is converted into a new current, called the "electric jet". An electrojet, among other things, heats and accelerates charged particles, turning them into a high-energy plasma stream.

Since the solar wind and the ejections of the coronal mass of the Sun are mostly protons and electrons, respectively, two types of auroras are distinguished.
Electronic auroras,
caused by electron flows and prevailing on Earth.
These are all familiar green or violet-raspberry arcs, radiant stripes, ribbons, curtains and other formations that have a fairly pronounced structure.
How are formed. - Electrons of the solar plasma, breaking into the upper layers of the Earth’s atmosphere, descend to heights of 400-100 km above sea level. Here, under their action, ionization of neutral atmospheric gases (oxygen and nitrogen) takes place, as well as the excitation of their atoms and molecules. In response to this, the molecules, atoms and ions of atmospheric oxygen and nitrogen emit light quanta at a strictly defined wavelength.

This determines the color of the auroras: for example, oxygen (the strongest line) is responsible for green, and nitrogen is responsible for purple, blue or red. In general, each aurora has its own unique palette of colors, depending on the constantly changing percentage of the chemical composition of the atmosphere.
Electron fluxes cause auroras on Earth, recorded not only in the visible range.
Rare on Earth, but still found only on Jupiter, are X-ray auroras.

The strongest X-ray aurora recorded on April 11, 1997 by the Polar orbiting satellite.
The picture shows x-rays (in conventional colors) generated in the upper atmosphere and caused by high-energy electron fluxes.
Proton auroras
It is also a rather rare phenomenon on Earth and its contribution to the glow of the Earth’s sky is relatively small.
Protons, falling into the Earth’s atmosphere, also collide with molecules and atoms of atmospheric gases, exciting and ionizing them. But at the same time, a proton can capture a free electron and a recharging process will occur. As a result, a neutral hydrogen atom is formed, which can emit photons in the visible and UV ranges.

The most common form of proton auroras is a rather wide arc, elongated in the direction from east to west, with a width of 300 to 1000 km. There are also arches and simply diffuse spots.

Red Proton Arch, Michigan

Powerful proton aurora in the UV range. Photo from IMAGE satellite
Now let's see how things are with the auroras on other planets.
Mercury
Everything is bad.
Despite the existing magnetic field, the intensity of which, however, is 100 times less than the Earth's, the atmosphere on the planet is virtually absent. It is so rarefied that the particles of the solar wind themselves make up the atmosphere of the planet, coupled with atoms knocked out of the surface. Atoms are more likely to collide with the planet than with each other.
Venus
Not so bad as it might seem.
The situation opposite Mercury is a dense and dense atmosphere and the absence of a global magnetic field. But despite this, Venus has a weak magnetosphere - it is induced by the solar wind itself, and not by the planet.
In the 2000s, Venus Express discovered that a magnetospheric tail, similar to Earth's, stretches behind Venus. In it, too, a reconnection of the magnetic field lines occurs. - Omnidirectional lines of a moving solar plasma are too close to each other and are closed.
The solar wind, controlled by the reconnection process, interacts completely with the atmospheric gases of Venus. Therefore, the aurora here is not entirely polar, or rather, not at all polar, and represents light and diffuse spots of various shapes and intensities. Sometimes they affect the entire planetary disk. Particularly visible on the night side of the planet.
Mars
There is no global magnetic field on Mars either, however, there is a residual local magnetization of the crust, especially in the highlands of the southern hemisphere.
The atmosphere of Mars is thin and rarefied, mainly consisting of carbon dioxide. When interacting with the electrons of the solar wind, which accelerates along the lines of local magnetic fields, rare and short-term ultraviolet auroras can be observed.
On August 14, 2004, the SPICAM instrument on board the Mars Express orbital station in the Cimmerian region recorded this phenomenon. The total size of the radiating region was about 30 km across, and about 8 km in height.

Local magnetic fields of Mars
But the proton auroras, first recorded during a solar storm on September 12-13, 2017 by the MAVEN orbiter, are unlike more powerful and global. They can cover almost the entire planet.
Mars is surrounded by an extensive crown of neutral hydrogen. The protons of the solar wind, which underwent the process of recharging in the corona, penetrate in the form of neutral atoms through the head shock wave (it only holds charged particles) and interact with atoms and molecules of atmospheric gases in the lower thermosphere (at altitudes of 110-130 km), generating ultraviolet radiation .
The radiation level on the surface of Mars, recorded during this solar storm by the Curiosity rover, broke all early records, almost twice exceeding their readings.
(Curiosity has such a device - "Radiation Assessment Detector" or RAD. It collects data to estimate the level of radiation background that will affect participants in future expeditions to Mars. The device is installed almost in the "heart" of the rover, simulating a person inside the space ship).
So during solar storms, the colonists on Mars are better off hiding somewhere.

Ultraviolet data is superimposed on the image of Mars on the night side before (left) and during (right) the event. Auroral radiation seems to be the brightest at the edge of the planet’s image along the line of the luminous atmosphere.
Gas giants
The four giant planets of the solar system have everything for the appearance of auroras - powerful atmospheres and strong magnetic fields.
An unpleasant feature of observations from the Earth (and generally from the inner regions of the Solar System) of the giant planets is that they face the observer with the side illuminated by the Sun. Therefore, in the visible range, their auroras are lost in reflected sunlight.
However, auroras in other electromagnetic ranges can be "detected". - UV radiation from hydrogen-rich atmospheres of giants is recorded by the Hubble Space Telescope. The X-ray range is again caught by the Chandra space telescope. And infrared detects even ground-based "Subaru".
Jupiter system
Need I say that the largest planet in the solar system has the most powerful auroras? In addition, unlike Earth, the auroras of Jupiter are permanent.
Another amazing feature of Jupiter’s aurors is that they arise not only due to the solar wind, but also due to the particle flows emitted by the planet’s satellites: Io, Ganymede and Europe (auroras are also observed on these satellites).
The presence of Io is particularly affected, since this satellite is volcanically active and has its own ionosphere.

Jupiter Aurora Borealis. Hubble combo shot, visible range and UV.
Io plays an important role in the formation of the magnetic field of the giant Jupiter. - Her volcanoes emit into the atmosphere a mass of ionized and neutral sulfur, oxygen, chlorine, atomic sodium and potassium, molecular sulfur dioxide, as well as dust of sodium chloride. All this substance is drawn by the magnetosphere of Jupiter from the thin atmosphere of Io at a speed of 1 ton per second.
In this case, depending on the ionization, this matter disappears either into a rarefied neutral cloud around the satellite (a yellow spot in the figure) or into a plasma torus surrounding the whole of Jupiter (the red region there).

Schematic of the Jupiter magnetosphere and the effects of Io: plasma torus (red), neutral cloud (yellow), flux tube (green) and magnetic field lines (blue)
And how does Io affect Jupiter’s auroras? It turns out that part of the ionized gas that the planet “steals” from its satellite is directed along the lines of force of the magnetic field to its poles (green vertical ring in the figure above). It turns out like a tube connecting Io and the polar regions of Jupiter, through which charged particles are pumped there. As a result, an “imprint” of Io forms in the atmosphere of Jupiter: an auroral spot, which follows the rotation of the satellite with some lag.

Animation created from images of the Hubble Space Telescope, spring 2005. Io's trail is visible on the right
In a similar way, but to a much lesser extent, Jupiter’s aurors are affected by two of its other moons - Europe and Ganymede. Their hot auroral spots are formed due to highly charged ions of oxygen, sulfur and, possibly, carbon, which actively exchange charges.

Auroral or hot spots (in ultraviolet light) of Io, Ganymede and Europe are traces of magnetic lines of force connecting the ionospheres of satellites with the ionosphere of Jupiter.
Bright spots inside the main rings, appearing from time to time, are believed to be associated with the interaction of the magnetosphere and the solar wind.

The Northern and Southern Auroras of Jupiter.
Photos of the planet and photos of the auroras taken by different instruments of the Hubble telescope (visible range and ultraviolet).
The X-ray auroras of Jupiter are extremely interesting. - Firstly, Jupiter is the only gas giant in the solar system that has X-ray auroras. Secondly, unlike the Earth, where the auroras at the north and south poles are almost a mirror image of each other, the radiation at the poles of Jupiter is “unsynchronized” - the south and north auroras behave independently of each other and change their intensity differently.
In addition, the x-ray of Jupiter pulsates. At the south pole - every 11 minutes, but at the north pole the aurora is unstable and changes its activity independently and with a different frequency - in different time periods - from 12 to 26 and even up to 40–45 minutes.
The reasons for such out of sync and ripple are still unclear.

X-ray auroras in the northern and southern hemispheres of Jupiter.
XMM-Newton and Chandra X-ray satellite data
And another question - how does Jupiter endow particles in its magnetosphere with huge energies necessary to create a constant stream of x-rays?
There is an assumption that the planet accelerates oxygen ions to incredibly high energies, which when colliding with the atmosphere at a speed of a thousand kilometers per second lose all eight electrons. Future observations of Chandra, XMM-Newton, and the Jupiter station Juno should reveal the nature of this process.
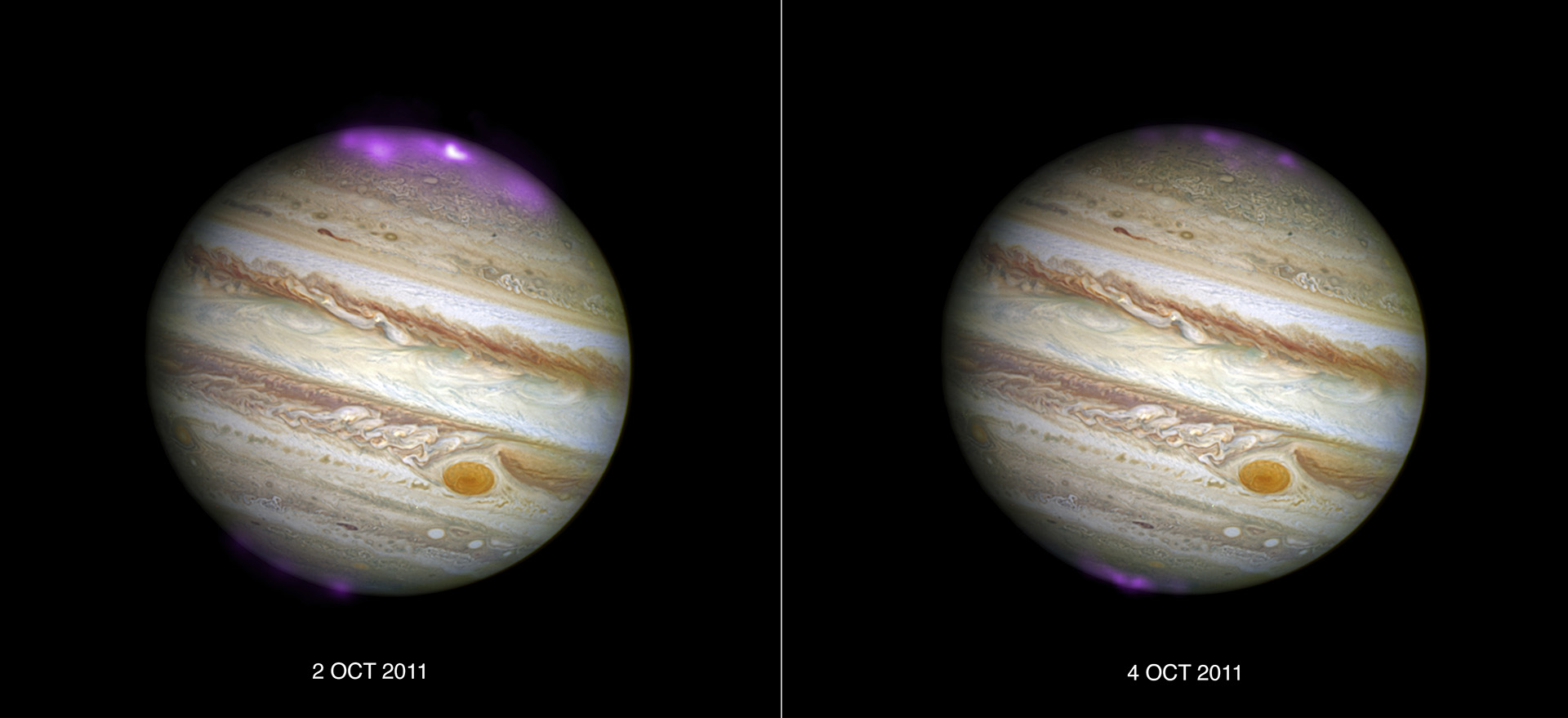
Hubble and Chandra X-ray combined photo

Photo + reconstruction of the aurora in the visible range above the north pole of Jupiter from the apparatus "Juno".
Orbital Jupiter station allowed to observe the dark side of the planet. December 18, 2018.

An infrared image of the aurora at the South Pole of Jupiter with the Subaru telescope.
The gases interacting with the solar wind in the upper atmosphere are heated, as on Earth. However, the heating of the Jovian atmosphere occurs two or three times deeper than on Earth, reaching the lower level of the stratosphere.
Well, one cannot fail to note Ganymede - the largest satellite in the solar system and the only one with its own magnetosphere. It is very small and immersed in the magnetosphere of Jupiter. However, the presence of Ganymede also has a weak oxygen atmosphere and cause the presence of ultraviolet auroras.
Observing the Ganymede aurora (and they depend on a change in the magnetic field of Jupiter - while the auroras on Ganymede seem to “sway”), scientists came to an amazing conclusion: under the Ganymede crust, a large amount of salt water is contained, and it affects its magnetic field.
The presence of the salty ocean creates a secondary magnetic field, which allows you to withstand the influence of Jupiter. This “magnetic friction” in some way suppresses the sway of the auroras. In practice, the swing of auroras is reduced to 2 degrees (instead of 6 degrees, which would be observed if the ocean did not exist).
According to scientists, the depth of the ocean is 100 kilometers, that is, it is about 10 times deeper than the oceans of the Earth. True, the Ganymede ocean is buried under a 150-170-km shell of ice.

Hubble combined photo in visible and UV ranges + Ganymede visualization.
Saturn system
Saturn also has auroras, where do they go.
Here Enceladus is “volcanically” active, the southern polar region of which actively spews fountains of water vapor with ice particles into the atmosphere surrounding the satellite. These emissions reach several hundred kilometers and even become part of the E ring, in which Enceladus rotates.
A part of this water vapor is ionized and in the volume of 100 kg per second replenishes the magnetosphere of Saturn with various hydro, hydrogen, oxygen and other ions and radicals.
However, they are not enough to inflate the giant magnetosphere to the size of the Jupiterian. Therefore, the auroras on Saturn depend much more strongly than on Jupiter on the intensity of the solar wind. In this they are similar to earthly ones.

Saturn’s Northern Aurora, captured by Cassini in the infrared (4 microns, blue). The clouds lying below are colored in conditional red (5 microns). A hexagonal cloud discovered earlier is visible right under the lights.
Auroras on Saturn, as well as on Earth, form closed or incomplete rings around the magnetic poles.
“The auroras on Saturn can be extremely volatile. - Now you see the whirlwind fireworks, and after a while you see nothing. In 2013, for example, we saw an unimaginable multitude of auroras on both poles of the planet - from steady bright rings to superfast flashes of light passing through the pole, ”draws Jonathan D. Nichols from the University of Leicester in England.

Saturn’s South Pole and the joint operation of the Hubble telescope in the UV range and the Cassini apparatus in the visible, infrared and radio ranges.
Three images of Saturn, taken at intervals of two days.

And Saturn in pure ultraviolet from Hubble.
Thanks to the ability of Cassini to observe objects in visible light, scientists were able to find out the colors of the auroras on Saturn. While the Aurors on Earth have green colors closer to the surface and red at the top, the probe's cameras showed that the auroras on Saturn have red colors closer to the center of the planet and purple in the upper atmosphere.
Particularly bright aurora on Saturn, shot at close range by the Cassini mission on November 29, 2010. The radiance goes down from the surface of the planet (occupying the upper part of the image) for 1400 km. Dashed lines indicate parallels and meridians, dashes at the bottom of the photograph are stars.

Uranus and Neptune
With Uranus, everything here is not thank God - it lies on its side, and the axis of the magnetic field does not pass through the geometric center of the planet. It “misses” by a third of the radius and is tilted by as much as 59 ° from the axis of rotation.
Greater "power" - only in Neptune. The axis of its dipole is shifted by 14 thousand km away from the center of the planet (this is 0.57 of its radii), and the center of the dipole is shifted by 6 thousand km to the southern hemisphere. Therefore, the magnetic field at the south magnetic pole is 10 times higher than at the north. But the slope is less - 47 °.
Despite such “curvature and oversizedness” of the axes, the magnetic fields of the ice giants are not so weak - Uranus almost like the Earth, Neptune only 2-3 times less. Accordingly, there are both magnetospheres and head shock waves, and auroras should be coupled with powerful atmospheres.
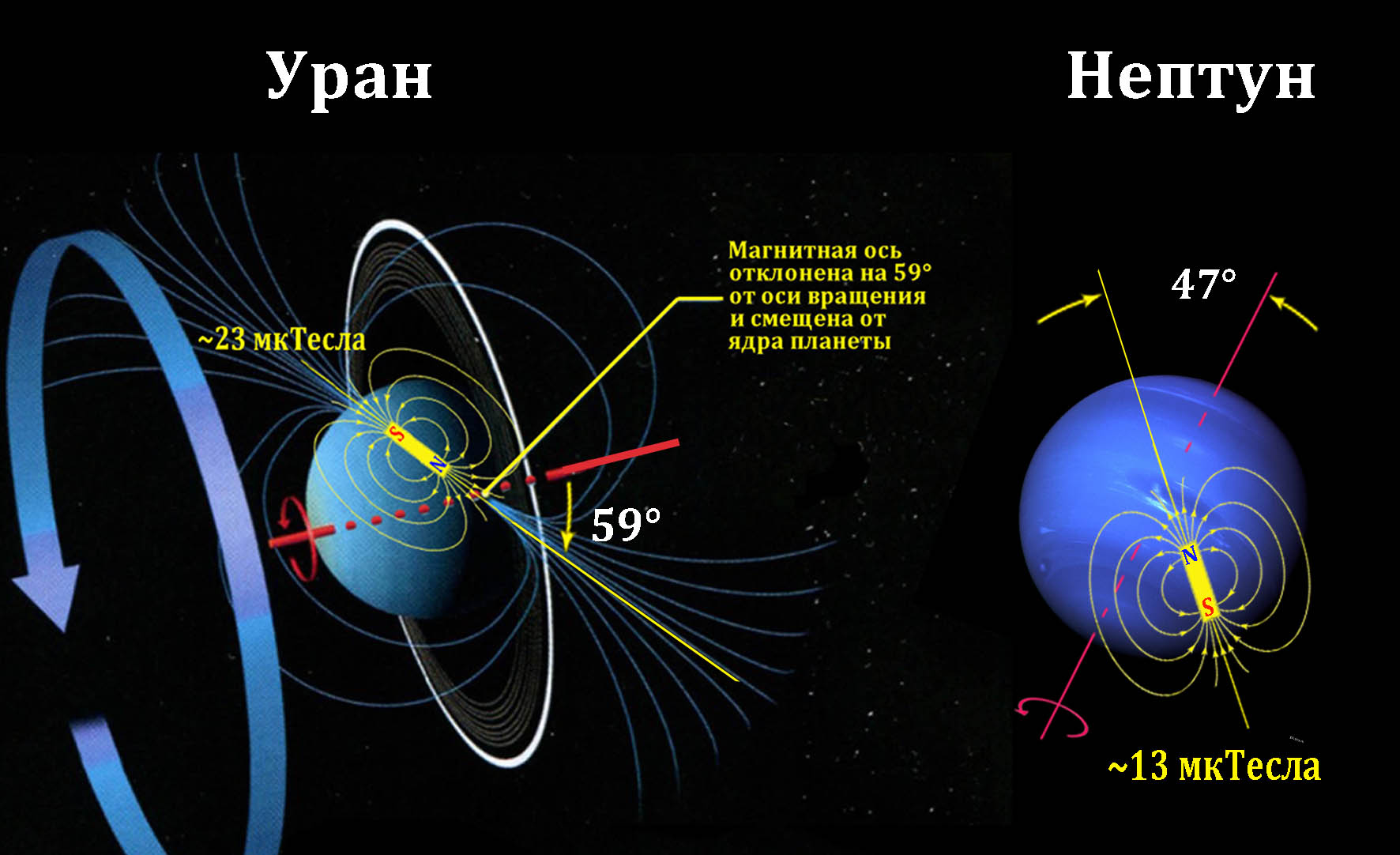
The difficulties of hunting for auroras on Uranus and Neptune are that it is not easy to calculate the “flight time” of the coronal ejection from the Sun. Even to the Earth, high-energy particles fly a day, two or three, and it is impossible to accurately predict the time. The ejection speed, of course, is known - it usually amounts to hundredths of the speed of light, that is, thousands of kilometers per second - but the result is also affected by the interaction of particles with the gravitational and magnetic fields of the sun.
It is easy to calculate that with an emission speed of 3000 km / s and a distance to Uranus of almost 3 billion km, the aurora on the planet will occur in about 11 days. However, the error in these calculations is large, and the operating time at the Hubble telescope is scheduled, so it is impossible to look at Uranus or Neptune for several days in a row.
And since the ice giants did not have orbital missions, in the case of Uranus, the first aurora was recorded only in 2011.
Aurora on Neptune has not yet been caught by telescopes. Voyager 2 observed auroras in the atmosphere of Neptune - they were scattered throughout the space (and not just in the oval areas around the poles). Aurors were also observed on Triton.

Uranus auroras captured by the Hubble UV spectrograph in 2011, 2012 and 2014.