A neural network that suppresses distracting information from the senses holds the keys to unraveling the work of attention and other cognitive processes.

We pay attention only to a small fraction of the information from the sense organs coming to us. New research results help to understand how the brain at each moment in time filters out the least interesting sensations.
We can listen to the conversation in a noisy room, among other voices becoming either quieter or louder, or amid the humming of an air conditioner. We can see a bunch of keys in a sea of trash, or a raccoon, rushing across the car. Sometimes, even with a huge amount of information filling our senses, we can concentrate on what is important to us and act accordingly.
Attention switching processes help the brain turn on the “spotlight” associated with the desired stimulus and filter out everything else. Neuroscientists want to identify the neural networks that guide and feed this spotlight. For decades, their research has been associated with the cortex, a wavy structure on the surface of the brain, usually associated with intelligence and the work of the mind. It became clear that the activity of the cortex enhances the processing of signals to concentrate on the things that interest us.
However, today some researchers are trying to use a different approach, studying how the brain suppresses information, and not how it complements it. More importantly, they found that the process involved more ancient and deeper parts of the brain - areas that are rarely considered related to attention.
Because of this, scientists unwittingly began to gradually move towards a better understanding of how deeply and inextricably intertwined body and mind - through the automatic perception of sensations, physical movements and higher-level consciousness.
Hunting for neural networks
The attention seemed so strongly connected with consciousness and other complex functions of the brain that for quite some time scientists considered it primarily a manifestation of the work of the cerebral cortex. For the first time, this idea was seriously abandoned in 1984, when Francis Crick, known for his work on DNA structures, suggested that the “spotlight” is controlled by a deep region of the brain called the thalamus , some of which receives data from the senses and transfers it to the cortex . He developed the theory that the sensory thalamus works not only as a transmitter, but also as a watchman - not only a bridge, but also a sieve - restraining part of the data stream to establish a certain concentration level.
However, decades have passed, and attempts to determine the mechanism responsible for this have failed - in particular because it is extremely difficult to study the attention of animals in the laboratory.
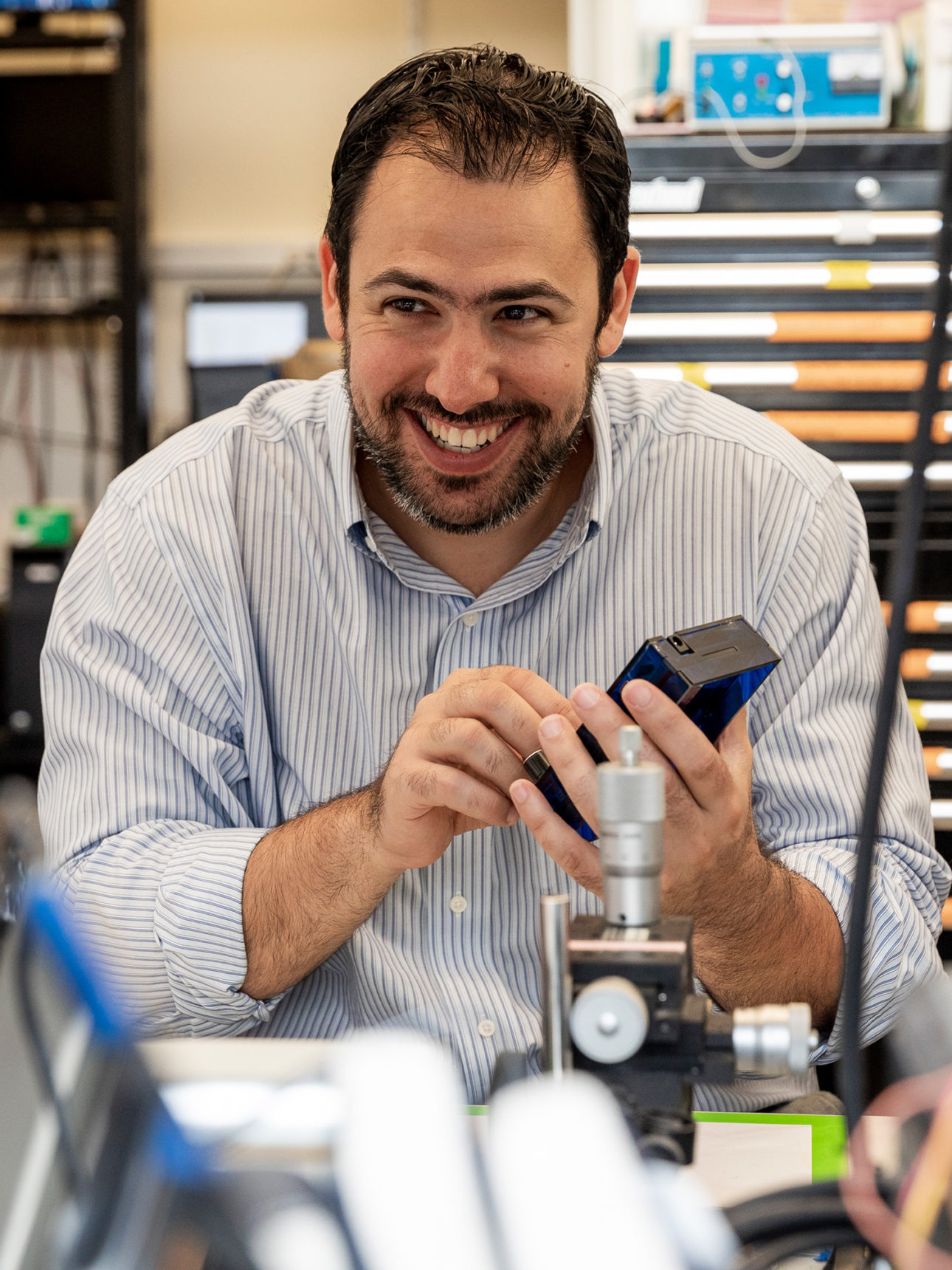
Michael Halassa, Neuroscientist at MIT
This did not stop Michael Halassa , a neuroscientist at the Brain Research Institute. McGovern at MIT. He wanted to determine exactly how the sensory data is filtered before this information reaches the cerebral cortex, to find a specific neural network, which, according to Crick, is engaged in this.
He was attracted by a thin layer of inhibitory neurons, the “reticulum nucleus of the thalamus” (SAT), which serves as the membrane of the thalamus. By the time Halassa became a postdock, he had already found an approximate screening mechanism: SNF was missing sensory data when the animal was awake, and paid attention to some aspect of the environment, but blocked them when it was sleeping.
In 2015, Halassa and colleagues found an even finer dropout rate, which allowed us to more reliably assign SNF to the long-wanted neural network - and this time it was related to how animals choose what to focus on when their attention is divided between several organs feelings. The study used mice trained to run as directed by flashing light and sound. Then the scientists turned on the animals simultaneously several conflicting commands from light and sound, while hinting to them which signal should be ignored. The response of the mice showed how effectively they were able to concentrate. In the experiment, scientists used well-established techniques for disabling activity in different parts of the brain to see in which cases this would prevent the animals from acting effectively.
As expected, the role of the prefrontal cortex, which gives high-level commands to other parts of the brain, was critical. However, the team also found that if in the experiment the mice needed to pay attention to vision, then disconnecting the neurons in the visual SNF prevented them from acting. Also, when these neurons were turned off, mice had difficulty concentrating on the sound. In fact, the neural network twisted the tuning knobs of inhibitory rather than exciting processes, and SNF inhibited information that the prefrontal cortex considered distracting. If the mouse had to give priority to auditory information, the prefrontal cortex gave a signal to the visual SNF to increase activity to suppress the visual thalamus, which turned off the flow of unnecessary visual data.
It turned out that the metaphor with attention as a searchlight works the other way around: the brain does not enhance the “lighting” of the stimulus of interest to it, but “dim the lighting” of everything else.
Despite the success of the study, scientists are faced with a problem. They confirmed Crick's suspicion: the prefrontal cortex controls the thalamus filter for incoming sensory information. However, the prefrontal cortex is not directly related to the sensory parts of the SNF. Parts of the neural network were missing.
Until recently. And then, finally, Halassa and colleagues put in place the last pieces of the puzzle, and the results revealed a lot about how to study attention.
We block, shade, blink
Using experiments similar to those they conducted in 2015, team members probed the functional interaction of various parts of the brain, as well as neural connections between them. They found that a complete neural network stretches from the prefrontal cortex to deeper structures, the basal nuclei (often associated with motor skills and many other functions), then goes to the SNF and the thalamus, and then returns to the higher regions of the cortex. So, for example, visual information coming from the eyes to the visual thalamus can be almost instantly intercepted if it is not related to the task being performed. The basal nuclei can intervene and activate SNF to filter external stimuli, in accordance with the instructions of the prefrontal cortex.
“This is an interesting feedback path that, in my opinion, no one has ever described before,” said Richard Krautslis , a neuroscientist at the National Eye Institute at the National Institutes of Health in Maryland, who was not involved in the study.
Moreover, the researchers found that the mechanism does not just filter out one feeling in order to increase attention to another: it also filters information within that one feeling. When mice were hinted that attention should be paid to certain sounds, SNF helped to suppress the background noise in the sound signal. The result of processing the input data from the sensory organs “can be much more accurate than simply suppressing the entire thalamus region for one sensory modality, which is a rather crude form of suppression,” said Duje Tadin , a neuroscientist at the University of Rochester.
“We often do not pay attention to how we get rid of less important things,” he added. “And I think there is a more efficient way to handle information.” If you are in a noisy room, you can try to raise your voice so that you can be heard - or try to eliminate the source of noise. Tadin studies the suppression of background effects in other processes that occur automatically, and also faster than selective attention.
Halassa’s discoveries suggest that the brain shuts off outside information earlier than thought. “What's interesting,” said Jan Fiebelkorn , a cognitive neuroscientist at Princeton University, “filtering begins at this very first step, before the information reaches the visual cortex.”
There is an obvious weak point in the brain’s ejection strategy for sensory information - namely, the danger that data thrown away may turn out to be unexpectedly important. Fiebelkorn's work suggests that the brain has a way to insure against such risks.
Fiebelkorn says that people imagine the spotlight as a constant beam of light, highlighting the places where the animal should direct its cognitive resources. “But my research shows that this is not so,” he said. “Apparently, this spotlight is blinking.”
According to his discoveries, the focus of the spotlight becomes weaker than four times per second, probably so that the animal does not concentrate too much in one place or one stimulus. This brief suppression of important information stimulates peripheral stimuli and creates the opportunity for the brain to shift its attention to something else if necessary. “The brain is apparently designed to be distracted periodically,” he said.
Fibelcorn and colleagues study subcortical regions with the goal of revealing the structure of neural networks. So far, they are studying the role of another section of the thalamus, but plan in the future, like the team of Halassa, to deal with the basal ganglia.
Thinking in action
These works mark a significant shift in neurobiology: once the processes of attention were considered the exclusive domain of the cortex. However, according to Krautslis, in the last five years "it has become a little more obvious that all this is happening under the bark."
“Most people want the cerebral cortex to do all the hard work, but I don’t think it is realistic,” said John Maunsel , a neuroscientist at the University of Chicago.
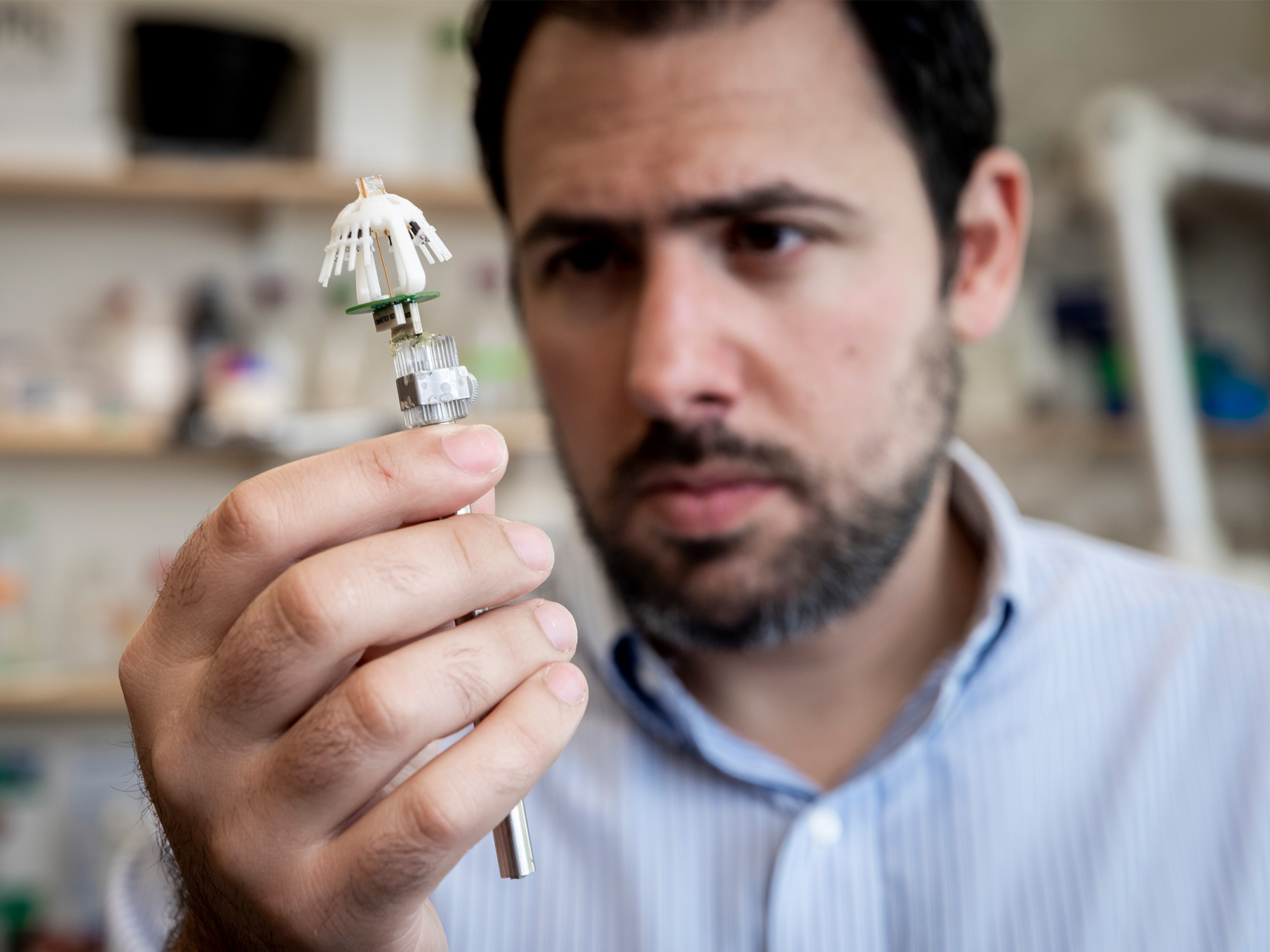
Halassa with a multi-electrode array, with which he and his colleagues controlled brain activity
The discovery by Halassa of the role of the basal ganglia in attention work is particularly interesting. In particular, because it is such an ancient part of the brain that was not considered as part of the network responsible for selective attention. “Fish also have it,” said Krautslis. “Up to the earliest vertebrates, including lampreys that don't even have a jaw” - or a neocortex - “they essentially have a simple version of the basal ganglia, and some of these same neural networks.” Fish neural networks can tell us how attention evolved.
Halassa is particularly interested in the fact that the connection between attention and the basal ganglia can open to us in the field of diseases such as attention deficit disorder and autism, which often manifest themselves as hypersensitivity to certain incoming sensory data.
But perhaps the most interesting of all, regarding the participation of the basal ganglia in these processes, is that this structure is usually associated with motor control, and some of the newer studies increasingly associate it with learning with rewards, decision making and other motivational types behavior.
After the work done in the laboratory of Halassa, the role of the basal ganglia was expanded to include the management of sensory data. This underlines the fact that “attention really consists in switching from one to the other in the correct order, during which you are not distracted by something that should not distract you,” said Maunsel. “The idea of including motility structures in this process makes sense - they should be at the very center of the decision-making process about what you will do in the next moment and on what you should concentrate sensory resources.”
This coincides with the growing popularity of attention to attention - and to thinking in general - as processes based on the so-called "Active findings." The brain does not just passively take information samples from the environment, responding to received external stimuli. The reverse process also takes place - such small physical movements as blinking also control perception. Sensory and motor systems "do not work separately, they evolved together," Fiebelkorn said. Therefore, the regions responsible for motility, not only help to shape the output parameters (animal behavior); they also help shape and input. Halassa's discoveries provide further evidence for such a proactive role.
“Perception is good for action, because we need to somehow imagine the world in order to act in it,” said Helin Slagter , a cognitive scientist at the University of Amsterdam. “And for the most part, we learn to perceive the world around us through action.” A large number of internal connections with the cerebral cortex suggests that in addition to controlling attention, "these subcortical structures play a much greater role in higher cognitive functions than is often considered."
And this, in turn, can give us ideas regarding consciousness, the most elusive topic of research in neurobiology. As follows from a study by Halassa and others, “when we study the correlation of neural connections and attention, in fact, we to some extent study the correlation of neural connections and perception,” said Maunsel. “This is part of a larger story, attempts to understand the principles of the brain.”
Slagter is now studying the role of the basal ganglia in the work of consciousness. “When perceiving the world, we do not just use our bodies, we feel it through bodies. And the brain builds a representation of the world in order to act meaningfully in it, ”she said. “Consequently, conscious perception must be closely connected with actions,” like attention. "Consciousness must be action oriented."