
The discovery of nuclear fission in the 1930s entailed the first threat of nuclear destruction through nuclear weapons in the 1940s, followed by the promise of clean and plentiful energy in the 1950s thanks to the advent of nuclear power plants. They had to replace other thermal power plants with one that does not produce exhaust gases, does not emit ashes, and requires only periodic refueling with uranium and other nuclear fuel, which can be found almost everywhere.

Equipment that first proved the possibility of experimental atomic fission in 1938
New nuclear reactors appeared with increasing speed in the 1950s and 1960s, which raised concerns about a possible shortage of uranium fuel, which led to an increase in the number of studies in the field of so-called “Fast neutron reactors”, which in their modification of breeder reactors can use uranium fuel much more efficiently. They use neutrons to convert (“multiply”) uranium-238 into plutonium-239, which can then be mixed with uranium fuel and create MOX fuel for slow neutron reactors, as a result of which, instead of 1%, one can use up to 60% of uranium energy.
The boom in uranium discovery in the 1970s halted research in this area, however, for example, France has consistently worked on its Rapsodie, Phénix and SuperPhénix projects, and only recently abandoned the 4th generation ASTRID technological demonstration after trying bring it to the end.
However, this is not the end of fast reactors. In this article, we will look at engineering miracles, and the different types of fast reactors that countries such as Russia, China, and India use or develop.
What is “fast” in fast reactors
Fast reactors makes the speed of neutrons in the process of nuclear fission. If in light-water reactors ordinary water is used to slow down neutrons, then this is not so in fast breeder reactors (BRR). The neutrons emitted by uranium-235 and other isotopes during a chain reaction move at significant speeds. Interestingly, the speed of the neutron determines the probability that it interacts with a specific nucleus.
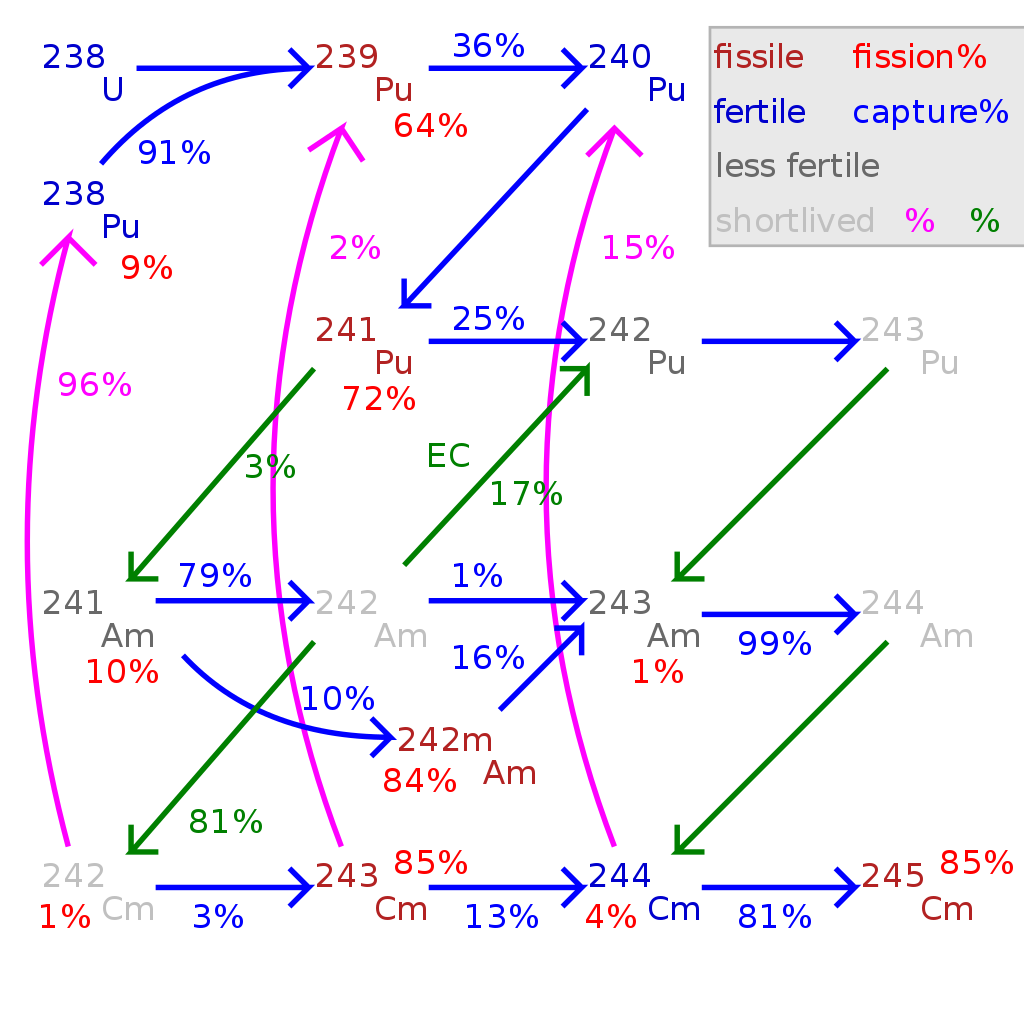
Production of transuranic actinides in thermal neutron reactors
To categorize nuclides, a property such as the neutron cross section is used . When a nucleus absorbs a neutron, and either saves it or decays, they say that it fell into the neutron cross section. Fissile nuclides have a fission neutron cross section. Other nuclides simply scatter neutrons - they have a scattering neutron cross section. Nuclides with large absorbing neutron cross sections are called "neutron poison", because they simply absorb neutrons without decaying, and, in fact, deprive the nuclear reaction of neutrons.
A nuclide of the uranium-238 type is interesting in the non-zero percentage ratio of each of these categories of neutron cross-section, which partially explains why it is so poorly suited as a fuel for light-water reactors. This is completely different in the case of uranium-235 - it has a large fission neutron cross section, but only at neutron speeds much lower than those possessed by neutrons released during a nuclear reaction. This means that neutrons in light-water reactors must be slowed down (to “temperature” speeds) to maintain the decay process.
And here between the fuel rods there is water, and neutrons fly everywhere here and there after the start of the decay process using the starting neutron source. These fast neutrons easily collide with hydrogen atoms in a water molecule, lose kinetic energy and slow down. As a result, they fly into another (or the same) fuel rod and successfully split another uranium-235 nuclide.
Also, this inhibitory property of water works as a safety measure. As the temperature in the core increases, the water boils and turns into a gas, because of which there will be fewer water molecules in the unit volume, the neutron deceleration will stop, and the speed of the nuclear chain reaction will drop. This negative steam reactivity coefficient is ubiquitous in modern reactors, with the exception of the notorious RBMK model and Canadian heavy water reactors CANDU .
We grow plutonium for fun and make money

Practically pure plutonium ring
As mentioned earlier, uranium-238 has rather strange characteristics of neutron cross sections. It both absorbs and scatters neutrons, and sometimes produces fission of the nucleus, and the first action occurs much more often. Having caught a neutron, the uranium-238 nuclide is converted (transmutes) to plutonium-239 (and some plutonium-239 nuclides are converted to plutonium-240). This process also takes place in a light-water reactor, but there it is going on intentionally - plutonium is made in this way in the BRR.
There is no neutron moderator in BRR, since it needs fast neutrons, which convert as much of uranium-238 as possible into plutonium-239. In BDS, the enriched core of uranium-235 is covered by a casing consisting mainly of uranium-238, which is slowly turning into plutonium-239 and plutonium-240, which are then used as MOX fuel. It turns out that the BRR operation scheme is relatively simple, and uses either a cooling circuit or a pool. As coolers, liquid metal or sodium-based refrigerant is usually used, since they weakly trap neutrons, but at the same time perfectly transfer heat.
French BRR were used both to generate electricity in the manner of conventional thermal power plants, and to produce plutonium, which is necessary to create MOX fuel, which can then be used in light-water reactors. The main reason for organizing this process was the desire for energy independence, since France does not have significant reserves of uranium. And such a process would make it possible to get up to 60 times more energy from imported uranium, which meant that each kilogram would last 60 times longer.
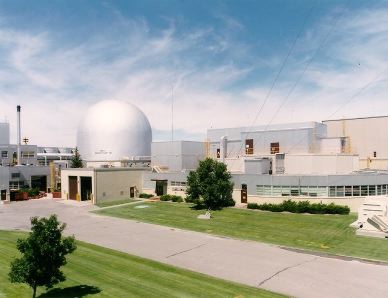
Experimental Breeder Reactor II (EBR II)
Other attempts to create fast neutron reactors include the fast integrated reactor in the United States and the Japanese Monju (followed by the Zoyo sodium-cooled fast neutron reactor). A pleasant side effect of the reproduction of uranium fuel is to save the amount of spent fuel at the end of the open fuel cycle, since the initially present uranium-238 is burned to plutonium-239 in a light-water reactor. The spent fuel can again be passed through the fast neutron reactor, where the “waste” of isotopes that cannot be used by the light-water reactor will be burned, and additional fuel will be created for the light-water reactor.
Unfortunately, RBDs are more expensive than light-water ones, and problems with sodium cooling (mainly the need to prevent contact with water) have led to the fact that since the fall in uranium prices in the 1970s it is usually more economical to create new fuel from uranium ore, and Store or dispose of used fuel after one open fuel cycle in a light-water reactor.
Despite the fact that the light-water reactor also multiplies fuel a little, converting uranium-238 into plutonium, its spent fuel still contains about 96% of the original uranium, about 3% of the “waste” isotopes, and about 1% of the plutonium isotopes.
Burn, baby, burn
Although most fast neutron reactors are used to multiply fuel for light-water reactors, another type is designed for local use of all fuel. Such a reactor is called a fast neutron reactor (RBN), and the configuration of its nucleus is different from the configuration of the BRR, but has no fundamental differences. Theoretically, any RBN can be used to reproduce and burn fuel.

Schematic diagram of a sodium-cooled fast neutron reactor
To change the scheme from RBD to RBN, you need to remove the uranium-238 coating and install neutron reflectors made of stainless steel (or something similar). In the resulting reactor, the released neutrons remain inside the nucleus, and can interact with nuclides, continuing the fission process.
As a result, RBNs can break down and convert nuclides in fuel until there is no significant amount of actinides left in it (including uranium and plutonium). This process can be combined with pyrometallurgical fuel regeneration, which allows reprocessing spent fuel in a light-water reactor for its use in the RBF, which essentially closes the nuclear fuel cycle.
French resistance
Not only the economy played a role in stopping the development of RBN in the West. The RBN attracted the attention of both terrorists and politicians. An example of the work of the former is the rocket attack on the Superphoenix nuclear power plant, carried out on January 18, 1982 by environmental terrorist Chaim Nissim. He fired at nuclear power plants from the RPG-7 Soviet hand-held anti-tank grenade launcher, believing that the RBN "could explode with all its fast neutrons." The nuclear power plant was a joint project of France, Italy and Germany, and it was originally planned to build the nuclear power plants of this project both in France and in Germany.

Superphoenix Reactor Building
From the very beginning, Superfenix faced strong political resistance from anti-nuclear groups, and the prototype of the reactor was closed in 1998, when the French government was led by green ministers. The only announced reason for the closure was that the project turned out to be unsustainable because of its "excessive cost", since 9.1 billion euros have been spent on it since 1976, that is, about 430 million euros per year. And this is despite the fact that in 1996 the problems with the sodium loop were solved, and the reactor really made money by supplying electricity for most of its existence.
Current developments
The situation in the USA, France and other western countries is very different from what was happening in the USSR, China and India. Since 1973, the BN-350 , located on the shores of the Caspian Sea (now this is the territory of Kazakhstan), provided the nearby city of Aktau with 135 MW of electricity and desalinated water. It was closed only in 1994, since the management company ran out of funds for the purchase of fuel. In 1999, after 26 years of operation, it was completely closed.
The BN-600 series was continued by the BN-600 reactor built at the Beloyarsk NPP in the Sverdlovsk Region near the city of Zarechny in Russia. It uses a sodium-cooled pool and has been operating since 1980, supplying 600 MW to the local grid. Despite several minor troubles, mainly related to sodium leakage, his work history was trouble-free, despite the fact that he was the second prototype in this series [from the moment the Phoenix reactor was shut down in France in 2009 until mid-2014 (launch BN-800) BN-600 was the only active fast neutron power reactor in the world / approx. transl.].
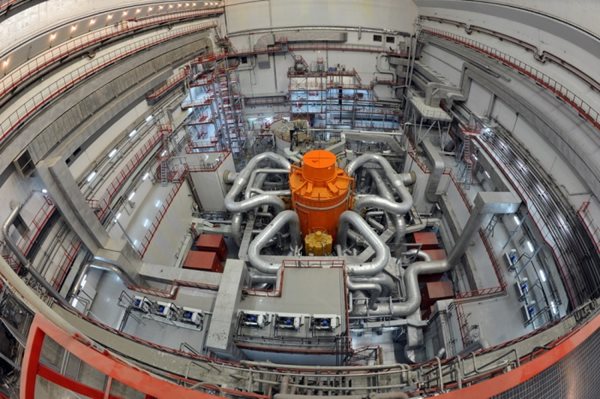
BN-800 in Beloyarsk
The BN-800 reactor, built in the same place in Beloyarsk, is the final prototype of the BN series and provides 85% service savings compared to the VVER-1200 light-water reactor. The designed BN-1200 will be the first mass production RBN. The Chinese experimental reactors CEFR FNR and CFR-600 are based on Russian BN reactor technology. Russia is also working on BREST with lead cooling BREST .
India discovered an abundance of thorium-232, which led to the creation of an ambitious program to develop thorium-based parallel to uranium reactors. The thorium program consists of three stages. First they produce plutonium from uranium using light-water reactors. The RBN then creates uranium-233 from thorium-232, burning plutonium. Finally, advanced heavy water reactors will have to use the resulting thorium as fuel, and uranium-233 and plutonium as auxiliary fuel.
Other IV generation RBNs are also being developed - for example, a gas-cooled fast reactor (HBR) with helium.
Closing the fuel cycle
As mentioned earlier, RBNs are capable of using all of today's spent fuel (which is often referred to as "nuclear waste"). Together with pyrometallurgical fuel recovery, this will allow nuclear fission reactors to work with virtually zero waste, using all uranium fuel, secondary actinides, and so on. This process is the main goal of the Russian nuclear program, and is also taken into account in the nuclear programs of China, Japan and South Korea.
In parallel with projects in the USA (mainly in the Argonne National Laboratory and its project for an integrated fast reactor with pyrometallurgical fuel regeneration), the Korean Atomic Energy Research Institute in South Korea is actively working to close the fuel cycle. The goal is to separate spent fuel from everything that is still suitable as fuel - that is, from what remains radioactive. Unfortunately, for political reasons, Russia practically does not work together on these projects with other countries, with the exception of China, and South Korea does not cooperate with anyone other than Japan and China.
But despite this, efforts continue to create IV generation RBNs and make it the preferred reactor for new nuclear power plants - this will allow not only the full use of reprocessed nuclear fuel and the closure of the fuel cycle, but also an increase in the amount of energy that we can extract from uranium (and, possibly thorium) many times. This will allow us both to increase even the most pessimistic estimates of the terms that we can hold out on existing uranium from hundreds of years to a comfortable few thousand, and not leave behind waste in the form of uranium fuel.