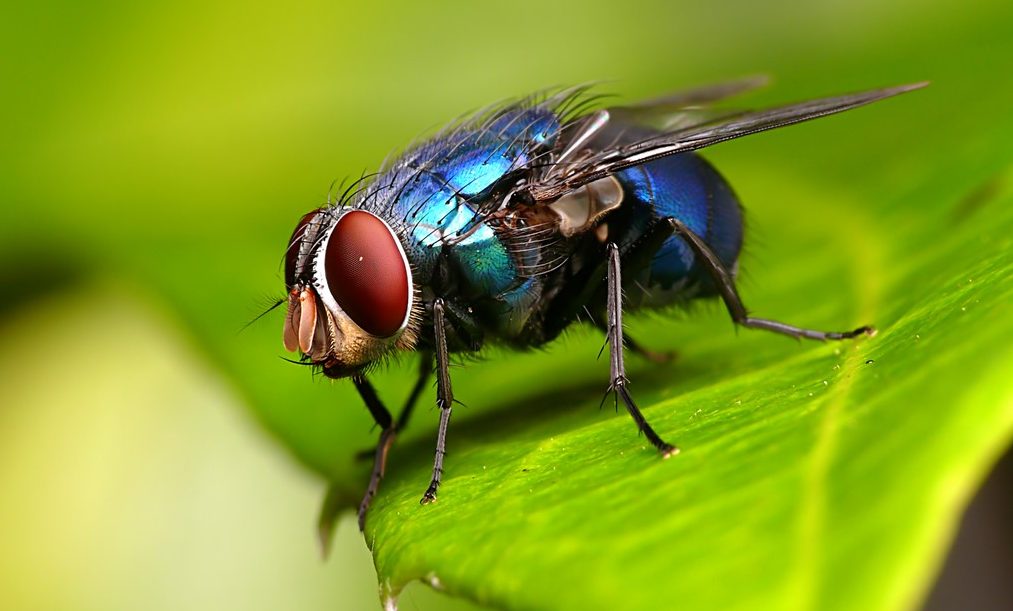
In August 1913, Peter Nesterov performed one of the most exciting, dangerous and complex maneuvers in aviation - the dead loop. At that time, especially considering the then technology, and now it is a real aerobatics. Many modern professionals and amateurs from the field of aviation would give everything to talk with Nesterov. Unfortunately, this is not possible, but other aces of aerobatics often live with us and often bother us. As a rule, we either don’t notice them, or we drive around the apartment with a slipper or newspaper, because for us they are pests, carriers of any infection and just annoying flyers. But for scientists, these creatures are the keepers of the secrets of flight and landing. Today we will meet with you an amazing study of the ability of ordinary flies to land upside down on any surface. What landing mechanism do flies use, what processes does it consist of, and how difficult is it to artificially implement it in robotics? We learn about this from the report of the research group. Go.
Study basis
Of course, you cannot give all the laurels of superiority in flying to flies, since many other insects also have their unique abilities in the field of flight and landing. However, the flies, as it were, accumulated in themselves the maximum number of such skills that are directly related to their anatomy.
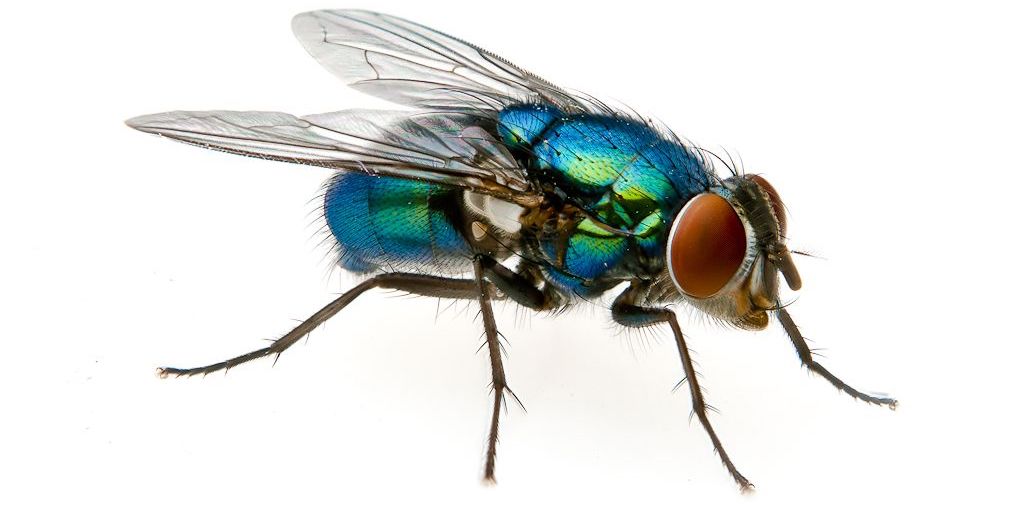
Appearance of a fly of the species Calliphora vomitoria.
In this study, the main role was played by flies of the species Calliphora vomitoria (blue fly). This species can be found in almost any corner of the planet: from South America to East Asia. The dimensions of these flies are quite impressive, 10-14 mm in length, which is approximately two times larger than that of ordinary house flies ( Musca domestica ). Externally, Calliphora vomitoria can be distinguished from its relatives by the blue abdomen, thanks to which it is also called the “ bluebottle fly ”, hinting at a resemblance to the color of the blue bottle glass.
In addition to the external differences from indoor flies, Calliphora vomitoria also has behavioral flies. Blue flies prefer to fly in a small swarm rather than singly, which allows them to quickly find food (usually this is the nectar of strongly smelling flowers). When one individual from the swarm finds food, it secrets a special pheromone, signaling the find to everyone else.
People often use larvae of blue flies as bait during fishing, but for insects this is not the most unique and, in truth, not the most worthy application. Not only fishermen find favor in the blue flies, but also the forensics. Flies, as we know, are not squeamish, because the corpses of animals and people do not scare them, but rather attract them. For forensic experts, this is an additional criterion for estimating the time of death, since blue flies have a very clear relationship between the growth rate of their larvae and the ambient temperature. Knowing the temperature, it is possible to determine from the larvae the time when the eggs were laid.
But today we will not talk about forensics, but about aviation. Inverted fit study, i.e. Landing on the ceiling, as scientists call it, was carried out earlier, but not in such detail. However, some interesting nuances were discovered. During observations of the inverted landing of house flies, scientists noticed that they make "semicircular" rotational movements before the contact of the ipsilateral * paws with the surface.
Ipsilateral * - located on the same side (exaggerated, the left arm of a person is ipsilateral with respect to the left leg).Following this movement, lateral oscillation of the body with the help of the legs occurred, which led to the contact of the remaining legs with the surface. These and other maneuvers before the actual landing, of course, are due to the sensor, i.e. visual, tactile and other signals received by the fly before landing.
In our study today, scientists from the University of Pennsylvania conducted a series of observations of the landing of blue flies on an inverted surface in a flight chamber (in a wind tunnel) using high-speed video ( 1A ).
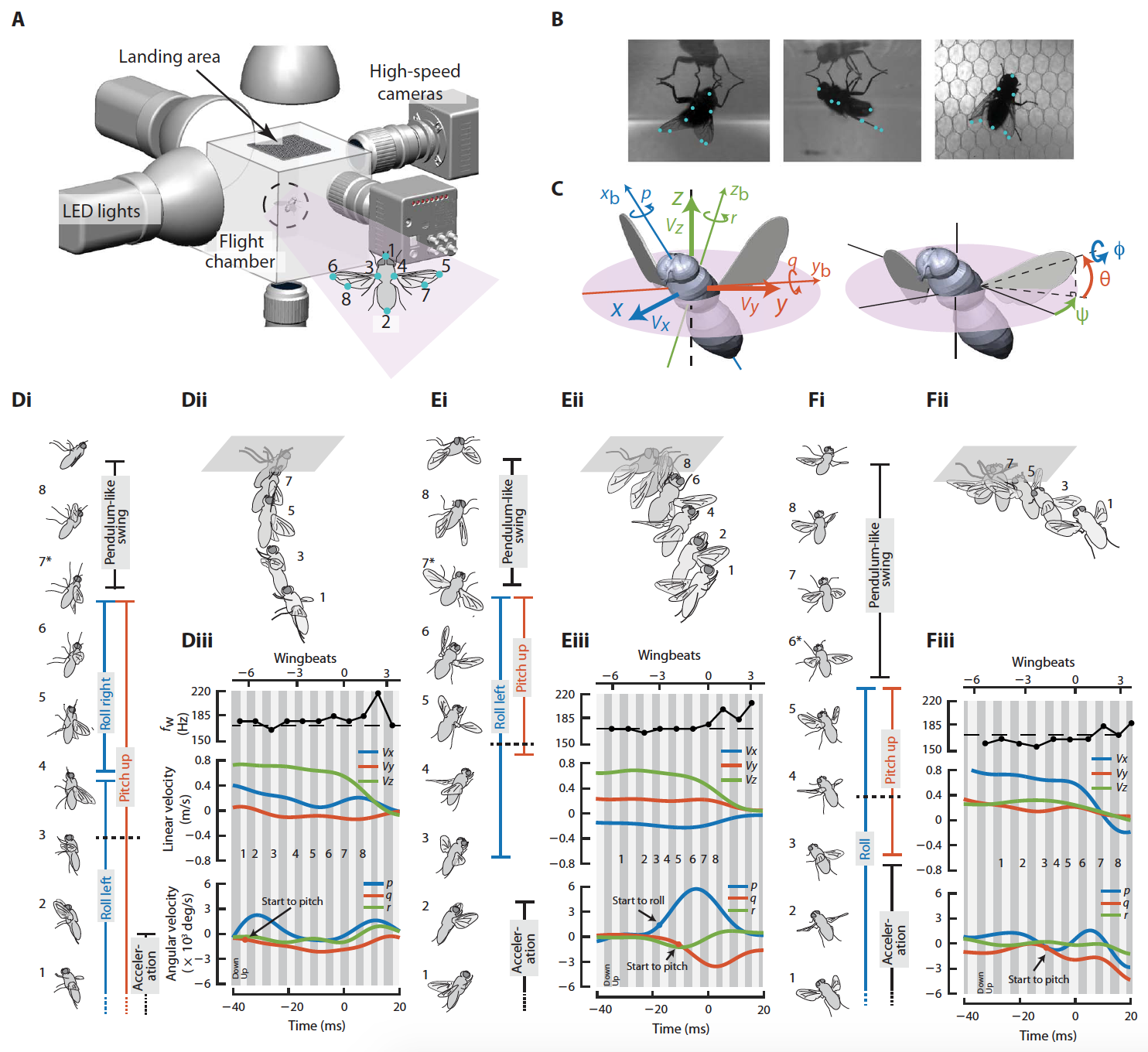
Image No. 1
The kinematics of the body and wings of flies were obtained by digitizing anatomical labels ( 1B and 1C ).
Observation results
First of all, scientists decided to isolate and analyze the behavioral aspects of a successful landing.
They note that fruit flies land on vertical surfaces through continuous deceleration and slight rotation of the body before planting. But the blue flies during landing showed immediately 4 consecutive behavioral aspects: vertical acceleration, rotational maneuver of the whole body, extension of the legs and swaying of the whole body with the front paws stably fixed to the surface. The duration of the entire successful landing process was approximately 4–8 wing impacts according to 18 observations, while the average wing impact frequency was 172.7 ± 7.7 Hz.
During successful landings, scientists also observed significant kinematic changes in the axes of rotation, the values of rotational maneuvers, and the degree of rocking of the body due to the paws.
Having gathered all the observations, the scientists identified the main strategies during the landing, which were classified as follows: dominant in pitch * , dominant in roll * , combined and dominant in lateral / longitudinal swing.
Pitch * - the angular movement of the body relative to the horizontal transverse axis.
Roll * - rotation of the body around its longitudinal axis.
Video # 1: landing with a prevailing pitch (corresponds to image 1D).
Video No. 2: landing with a prevailing roll (corresponds to image 1E).
Images 1D - 1F show typical patterns of behavior of a blue fly during landing. In the first two examples, flies used fast rotational maneuvers, mainly relative to the axis of pitch ( 1D and video No. 1) or roll ( 1E and video No. 2), which set their body before landing in an almost inverted (belly up) orientation.
The alignment of the paws occurred almost immediately after the start of the rotational maneuvers (horizontal black dotted lines on 1Di and 1Ei ). After the paws touched the surface, the body rocking phase began.
It is curious that the average angular velocity of rotational maneuvers reached approximately 4000 ° / s, and its maximum value could exceed 6000 ° / s, which is significantly higher than that of fruit flies or even hummingbirds.
Video # 3: landing with the prevailing longitudinal vibration of the body (corresponds to image 1F).
In the third version, the flies relied almost entirely on the oscillation of the body with their paws ( 1F and video No. 3), and the rotational maneuver was negligible.
Video # 4: landing with prevailing lateral body sway.
Thus, compared with the first two options, this landing strategy probably used more mechanical and structural processes (for example, adhesion * due to pulvillas * or damping * due to viscoelasticity of the paws), which facilitated the transmission of the linear momentum of the body to the rotational momentum .
Adhesion * - adhesion of surfaces of different origin (for example, dew drops on grass).
Pulvilla * - soft pads of the tip of the legs, each of which is located under its claw. The main task of pulvillas is to keep the insect on a smooth or inclined, vertical surface.
Damping * - artificial suppression of vibrations.The next step in the study was to study the kinematic differences between successful landings and unsuccessful ones.
Video No. 5: landing with combined roll-pitch mechanics.
Unsuccessful landings included those that ended in a collision with the surface (which is for scientists the data for analysis, then for the flies a concussion, figuratively speaking, of course).
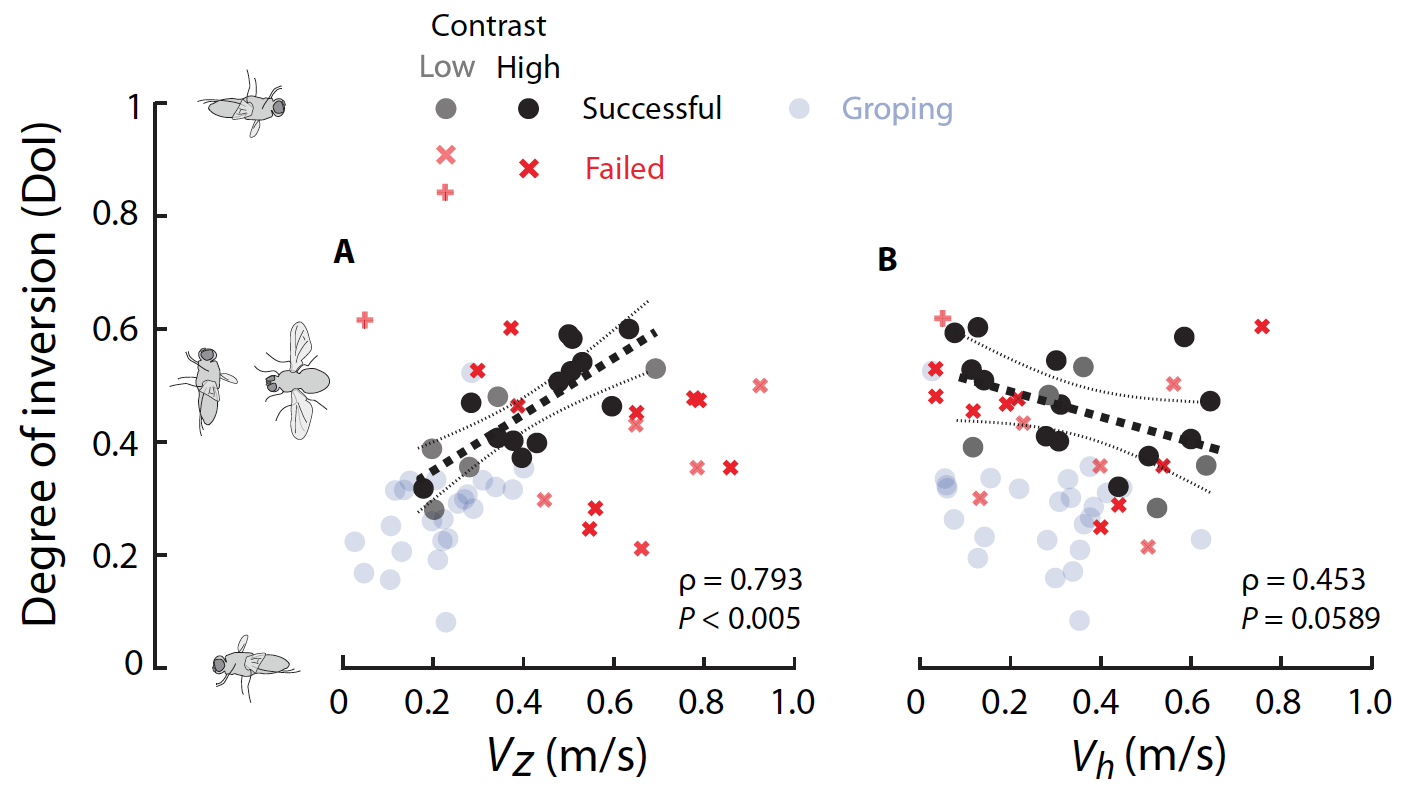
Image No. 2
To quantify how well the fly body was oriented, a degree of inversion (DoI from degree of inversion ) was calculated, which measures the degree to which the fly body is aligned relative to the fully inverted orientation before landing. DoI varies from 0, which means the absence of inversion of the body, i.e. abdomen down to 1, which means inversion of the whole body, i.e. belly up. On successful landings, DoI increased at a vertical speed ( 2A ), but decreased at a longitudinal speed ( 2B ).
This observation indicated that the flies needed to roll over more when their ascending speed was higher or when their horizontal speed was lower. In the majority of unsuccessful landings (there were 15 in total), the flies were not turned upside down before the moment of landing compared to the position of the flies that made successful landings.
The cause of failure could be untimely or insufficient rotation of the body before landing. Thus, these flies could not properly land on the ceiling, but crashed into it.
Video # 7: failed landing due to rotation too early.
In addition, unsuccessful landings also occurred due to too early rotation of the body (video No. 7), since an earlier inversion led to insufficient vertical speed necessary to reach the ceiling (marked with a “+” on graphs 2A and 2B ).
Video No. 8: unsuccessful landing due to weak inversion of the body with untimely alignment of the legs.
Another reason for the failures, despite the correct inversion and speed of movement, was the late alignment of the paws, which led to an incorrect location and, therefore, insufficiently tenacious contact with the surface (video No. 8).
Video # 6: an example of contact landing on the ceiling.
Despite all the punctures and shortcomings, some flies could still recover and land successfully using a contact landing. In this case, the flies fly with a low vertical speed under the ceiling, then they feel the surface using the front paws, and then they cling and land (video No. 6).
Video No. 9: unsuccessful landing due to weak inversion of the body with slight rotation of the body.
Summing up the data obtained from observations, scientists came to the conclusion that the kinematic aspects of the landing of the fly contain much more different neural processes than previously thought. The most striking feature is still the rotational maneuver. It remains only to understand what provokes it, or rather what signals lead to the fact that the fly uses it for landing.
The first to come to mind, of course, are the visual signals that the flies receive when approaching the ceiling with a linear speed consisting of three components ( 1C ): vertical ( V z ), longitudinal ( V x ) and lateral ( V y ).
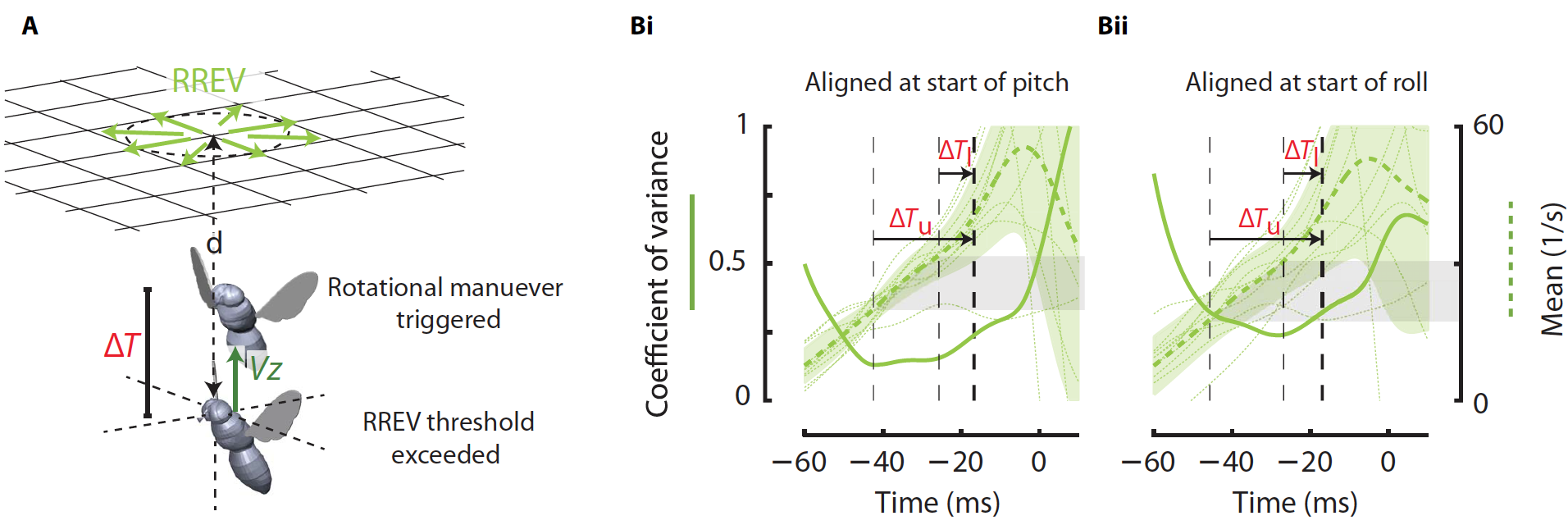
Image No. 3
Thanks to the work of their predecessors, researchers accurately know the three main types of visual cues needed to evaluate fly movement.
First, the relative retinal expansion rate ( RREV ) due to immobile stimuli ( 3A ). This indicator can be calculated as the ratio of the rate of increase of the target to its actual size on the retina of the fly during upward movement ( V z ). RREV also corresponds to the inverse of the time before the collision ( t ). RREV is considered an important signal of perception, which controls the speed of approach during landing or walking around obstacles.
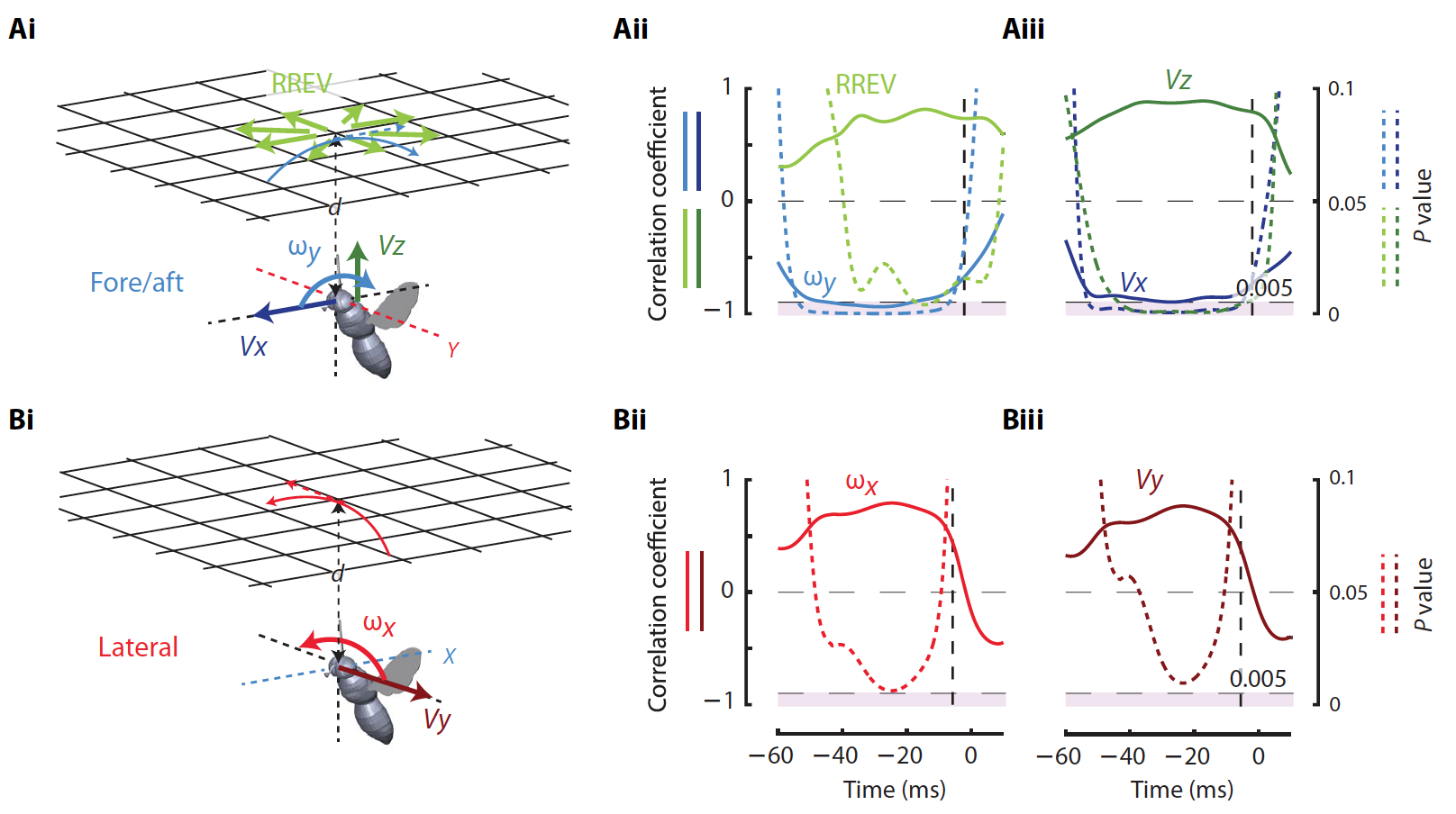
Image No. 4
Secondly, the relative angular velocity of the longitudinal direction ( 4Ai ) of the ceiling on the fly retina (ω y ). These visual cues are the result of moving the body forward / backward ( V x ), i.e. in the horizontal direction. It was previously found that it is these signals that regulate landing on horizontal surfaces.
Thirdly, the relative transverse angular velocity of the ceiling (ω x ) on the fly retina ( 4Bi ) obtained as a result of lateral displacement ( V y ).
The researchers suggest that the rotational maneuver starts after a fixed time delay when the provocative (signal, which leads to the actual action) visual signal reaches a threshold value. This assumption allows us to evaluate the relationship of the above signals and the maneuvers of movement performed by the fly during landing.
The first step was to calculate the time curves of the coefficient of variation (CV) of each of the visual signals. The observations were taken as the basis, during which the flies successfully landed on the ceiling on the first attempt.
If rotational maneuvers were started after some delay, when the visual signal reached a threshold value, the CV of this visual signal should be minimal. In addition, it should be lower than other non-provocative visual signals, i.e. provocative visual signal should have the smallest dispersion. Observations and calculations showed that among the three tested signals, it was RREV that had the lowest CV value at the moment of turning the turn (15%) and roll (25%), while the other two visual signals had significantly higher CV ( 3B ).
This means that RREV is the main visual signal that triggers the start of a rotational maneuver during an inverted landing.
The CV value for RREV was minimal between 7 ms (∆T l ) and 27 ms (∆T u ) before the start of the rotational maneuver. Consequently, the visual delay DT between the moment of perception and the beginning of maneuver was in this small range. It was also found that the critical time before a collision, below which the rotational maneuver starts (to avoid a collision and successfully land), is 31-53 ms. For comparison, for flies landing on vertical surfaces, this figure is 76 ms. In other words, when landing on the ceiling, flies have significantly less time for error.
Rotational maneuvers, judging by the observations, were quite variable in terms of roll speed and pitch. This variability may indicate that the rotational maneuver is regulated not only by the time component, but also by other sensory signals.
Flies use sensory signals to provide rotational maneuver through the direct and feedback channels. The direct communication channel issues spontaneous control commands that cause significant transient changes in the movement of the wing. These changes directly affect the amplitude of the primary reaction to the maneuver.
The feedback channel provides a compensatory response that causes a small change in the movement of the wing. Such changes affect damping (vibration suppression) and the stabilization of maneuver.
The degree of influence of a particular sensor signal on the maneuver was assessed by revealing the correlation of the maximum roll and rotation speed during the rotational maneuver with the sensory signals received by flies before or after the start of maneuvers.
The proposed sensory signals include three visual (RREV, ω x and ω y ), discussed earlier, and three components of the linear velocity ( V x , V y and V z ).
Linear regression analysis showed that for a long period of time before the start of maneuvers, the maximum pitch speed was positively correlated with RREV ( 4Aii ) and the vertical speed V z ( 4Aiii ), but negatively with the longitudinal rotation ω y ( 4Aii ) and the longitudinal linear speed V x ( 4Aiii ).
In turn, peak roll velocities positively correlated with lateral rotation ω x ( 4Bii ) and lateral linear velocity V y ( 4Biii ) during an extended period of time before and after the start of maneuver.
The analysis also indicated that flies can change the strength of the rotational maneuver in order to more effectively use the body vibrations at the time of landing, i.e., the transmission of a linear impulse to an angular one.
As observations showed, the pitch velocity decreased when the linear pulse was high, as indicated by the negative correlation between the peak pitch level and the sensor signals. Thus, instead of pitch, the flies turn their body over, increasing the longitudinal oscillation of the body with their paws and transmitting a direct linear impulse to the pitch angular momentum.
An increase in roll speed was also observed when the lateral linear impulse was high, as indicated by a positive correlation between peak roll speed and sensory signals. Such an inverse correlation can occur in situations where flies need a greater distance to raise their ipsilateral paws high enough and reach the ceiling for further rocking of the body.
Do not forget about the most important attribute of flights for flies, i.e. about wings. The researchers analyzed the kinematics of the wings of flies during a landing on the ceiling, since it is the wings that create the various aerodynamic impulses necessary for successful maneuvers.
Observations of the wings of blue flies revealed a number of changes occurring at the time of the maneuver.
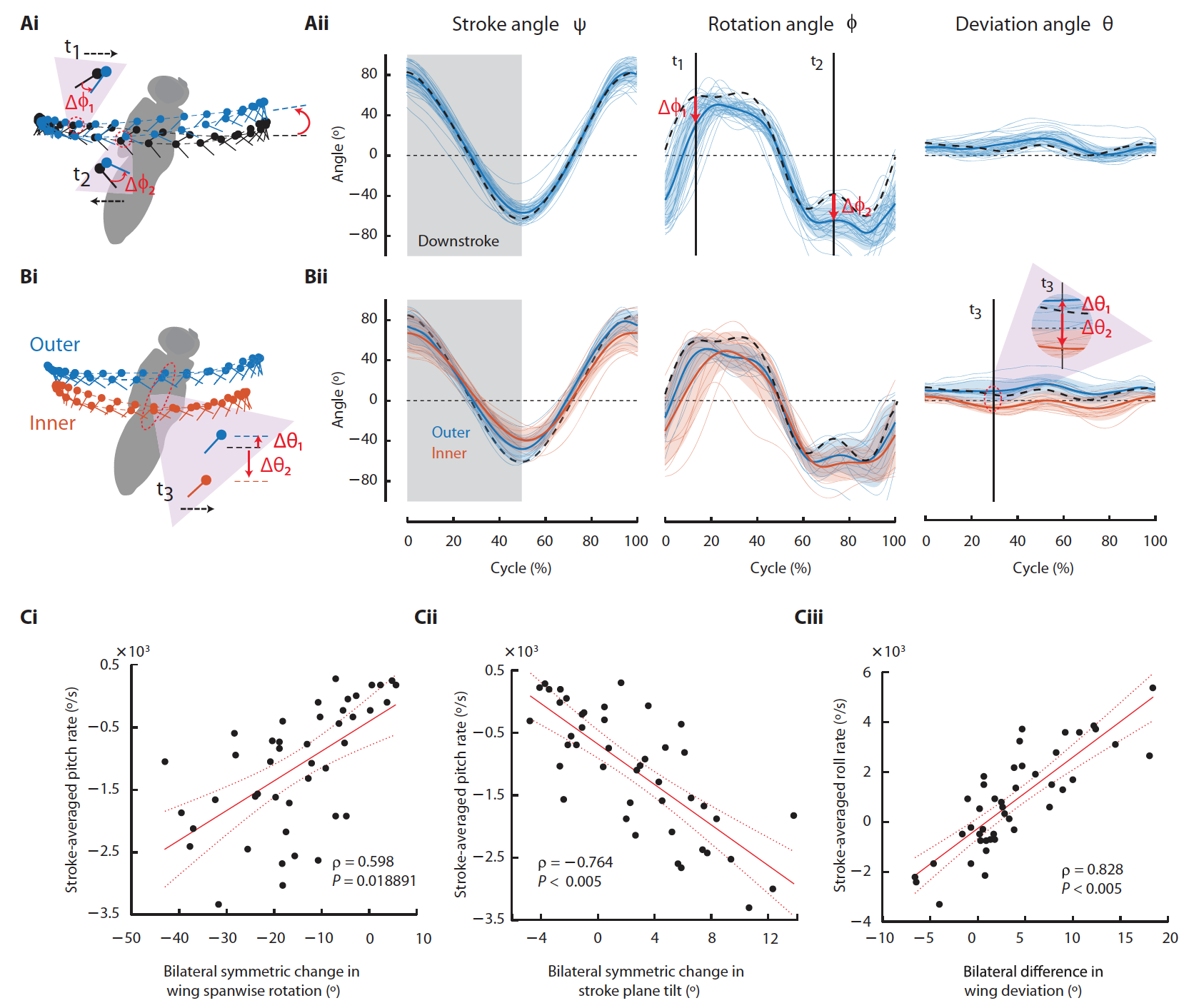
Image No. 5
The first thing that was discovered was a bilateral symmetric change in the longitudinal rotation of the wing ( 5A and 5Ci ) and bilateral symmetrical changes in the angle of the plane of the wing ( 5A and 5Cii ), which both strongly correlated with the pitch frequency. There were also changes in wing tilt ( 5B and Ciii ), which strongly correlated with roll speed. In addition, there were also changes in the amplitude of the flapping and rotation of the middle wing, which contributes to the roll.
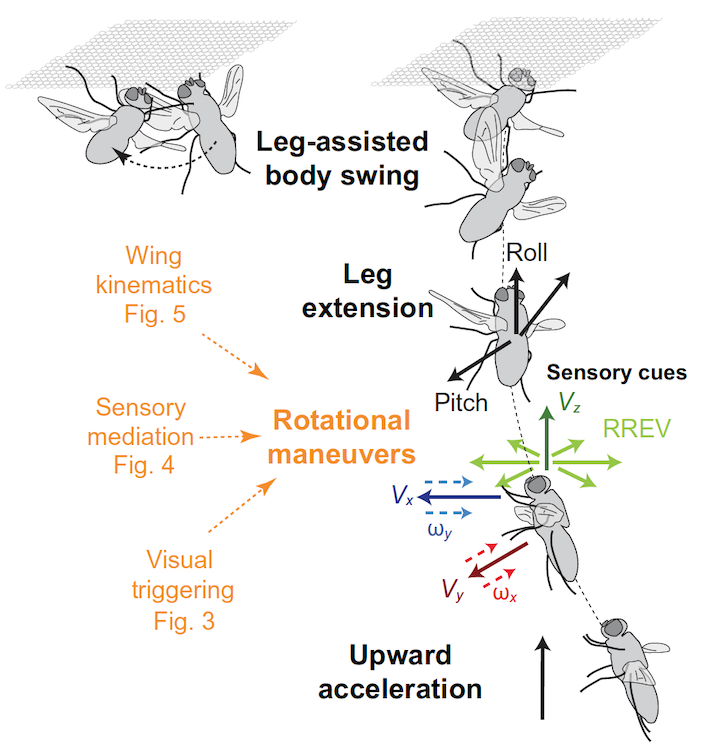
Image No. 6
For a more detailed acquaintance with the nuances of the study, I recommend that you look into the report of scientists and additional materials to it.
Epilogue
Summing up all the observations and calculation results, the researchers concluded that the inverted landing on the ceiling is due to a well-coordinated sequence of behavioral modules (diagram above).
Landing on the ceiling can include neural processes that not only compute RREV encoding information about the time before the collision, but also other sensory signals that are supposed to encode multi-axis linear movement of the body.
At the moment, scientists still can not accurately answer the question of which sensory signals lead to certain movements during the maneuver. But the work carried out unequivocally provides the basis for future research.
Observations of insects and birds have always served as inspiration for man to create aircraft. Studying blue flies is no exception. Scientists believe that their observations indicate that for the successful implementation of a robotic or manned vehicle capable of landing on inverted surfaces, a tight integration of computing and mechanical processes is required. For example, it can be a combination of quick recognition schemes for images and a system for controlling the position of the device plus a stabilization system that flies realized by vibrating the body on their legs.
Once a man could only dream of flying, now it is a reality. But we are only guests in this environment alien to us, which is native to many living organisms, and we still have a lot to understand, discover and explore before we become their full-fledged neighbors in the clouds.
Thank you for your attention, remain curious and have a good working week, guys! :)
Thank you for staying with us. Do you like our articles? Want to see more interesting materials? Support us by placing an order or recommending it to your friends, a 30% discount for Habr users on a unique analog entry-level server that we invented for you: The whole truth about VPS (KVM) E5-2650 v4 (6 Cores) 10GB DDR4 240GB SSD 1Gbps from $ 20 or how to divide the server? (options are available with RAID1 and RAID10, up to 24 cores and up to 40GB DDR4).
Dell R730xd 2 times cheaper? Only we have 2 x Intel TetraDeca-Core Xeon 2x E5-2697v3 2.6GHz 14C 64GB DDR4 4x960GB SSD 1Gbps 100 TV from $ 199 in the Netherlands! Dell R420 - 2x E5-2430 2.2Ghz 6C 128GB DDR3 2x960GB SSD 1Gbps 100TB - from $ 99! Read about How to Build Infrastructure Bldg. class c using Dell R730xd E5-2650 v4 servers costing 9,000 euros for a penny?